Cloning Enzyme Resource Guide
クローニング
Cloning Enzyme Resource Guide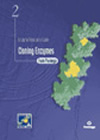
クローニングに使用される酵素類の説明やプロトコルなどが記載されています。
英語版(PDF) | オンライン閲覧 |
---|
Subcloning Guide An introduction to fundamental techniques for DNA transfer between vectors, including restriction digestion, PCR cloning, dephosphorylation, and bacterial transformation. This guide provides a comprehensive introduction to basic subcloning, including example protocols. For ordering information on the products discussed here, visit the Cloning and DNA Markers product listings. View Products Subcloning Strategies Subcloning is a basic procedure in molecular biology for transfer of DNA inserts from one vector to another to gain functionality to study the sequence of interest. Essentially all subcloning reactions proceed the same way as illustrated in the figure below. You release and purify your insert from the parent vector, ligate this insert into a prepared destination vector, and transform this ligation reaction into competent bacterial cells. Then, you screen the transformed cells for the inserted DNA. Before you begin your subcloning experiment, you need to know the which restriction enzyme (RE) sites are present in the multiple cloning sites (MCS) in both your parent vector and destination vector, and if these same sites also occur in your insert. Once you know this information, you can begin to develop your subcloning strategy. Subcloning overview diagram Subcloning Strategy: Common Restriction Sites If the multiple cloning sites on both the parent and destination vectors contain two restriction sites in common that do not occur within your insert, you have a very straightforward subcloning process. You digest the parent and destination vectors with the two enzymes they have in common, followed by dephosphorylation of the destination vector. The insert and the dephosphorylated vector are then separated on an agarose gel, purified using a system such as the Wizard® SV Gel and PCR Clean-Up System, and ligated. Restriction digestion with compatible ends. The T4 DNA Ligase will join the DNA by reforming the bond between the 5´-PO4 coming from the insert and the 3´-OH of the vector. The vector has been dephosphorylated so the second bond will not be formed in vitro (indicated by the OH). These nicks will be repaired in the bacteria upon transformation. Having a restriction site in both the multiple cloning region and the insert does not exclude the use of this site for subcloning. Partial Restriction Digestion can be performed. Subcloning Strategy: Common Restriction Sites with Partial Digests Controlling Cut Frequency in Restriction Digestion The presence of the same restriction enzyme recognition site in the insert and the multiple cloning region does not necessarily preclude use of that restriction site in a subcloning strategy. Under normal restriction digest conditions, the enzyme is in excess so that all recognition sites in the plasmid can be cleaved. You can manipulate the restriction digest conditions such that you will digest only a subset of sites. Many strategies have been employed to do partial digests including decreasing reaction temperature, using a non-optimal buffer, and decreasing units of enzyme. 4500ma Subcloning Strategy: Moving Inserts with Compatible Restriction Sites Even if you don’t have common restriction sites in the parent and destination vector multiple cloning regions, you may have compatible restriction sites. Compatible restriction sites have the same overhang sequence and can be ligated together. In this example, XbaI and NheI both produce the same 5´overhang sequence. Cut sites from these two enzymes are exactly matching and ligate well. However, neither the XbaI nor NheI sites are regenerated during ligation. Compatible end ligation is straightforward after the enzymes are identified. 4501ma In this example, none of the restriction sites used for the compatible-end subcloning are regenerated in the final ligation product Subcloning Strategy: Moving Inserts with Only One Common Site You’ve looked for common sites or compatible sites and you can find only one match on one side of your insert. What should you do about the other side of the insert? Make it a blunt end using T4 DNA Polymerase or the DNA Polymerase I Klenow Fragment! Simply digest the parent vector with your chosen restriction enzyme and use T4 DNA Polymerase or the DNA Polymerase I Klenow Fragment to make the end blunt. Run the products on a gel, purify the desired fragments, and proceed with the common or compatible end restriction enzyme digestion of the destination vector. In this example, the destination vector has a SmaI site, which leaves a blunt end. Most vectors have at least one blunt-ended restriction site that can accept the newly created blunt end of the insert. If you don’t have such a site or the site would not be in the correct orientation, the same blunt-end creation strategy may be applied to the destination vector as well. 4502ma Subcloning Strategy: Blunt-End Method You can’t find a single common site or compatible site in the parent or destination vector. What do you do? Many people resort to amplifying the insert with restriction sites in the primers to provide the compatibility, but this strategy may lead to more problems including introduction of mutations or difficulty digesting PCR products. You could try straight blunt-end cloning. Cut out your insert from the parent vector and open the destination vector with whichever enzymes you desire. Purify the insert. Treat both the purified insert and opened destination vector with T4 DNA Polymerase or the DNA Polymerase I Klenow Fragment. Remember, this method will not retain the orientation of your insert so if orientation is important, you will have to screen for orientation. 4503ma Restriction Digestion Restriction endonucleases (RE), also referred to as restriction enzymes, are proteins that recognize short, specific (often palindromic) DNA sequences. Type II REs cleave double-stranded DNA (dsDNA) at specific sites within or adjacent to their recognition sequences. Many restriction enzymes will not cut DNA that is methylated on one or both strands of the recognition site, while some require substrate methylation. Restriction digestion is one of the most common reactions performed in molecular biology. For a digestion with a single RE the reaction is very simple: Nuclease-Free Water 14µl 10X Restriction Buffer 2µl Acetylated BSA (1mg/ml) 2µl DNA (~1µg) 1µl Restriction Enzyme (10u) 1µl Final Volume 20µl Mix by pipetting and collect the contents at the bottom of the tube. Incubate at the appropriate temperature and the appropriate time for the enzyme. Add 4µl of 6X Blue/Orange Loading Dye and analyze digested DNA by gel electrophoresis. Preparing an insert for transfer from one vector to another usually requires digestion with two different REs. This is called a double digest. If both restriction enzymes work in the same restriction enzyme buffer, the reaction is straightforward. Simply add 1µl of the second restriction enzyme and adjust the amount of water used to maintain a 20µl reaction. Remember, restriction enzymes are commonly stabilized in 50% glycerol solution. Do not exceed 5% glycerol in final digest with the two enzymes. Glycerol concentrations >5% may lead to enzyme star activity, which means the enzyme will lose sequence specificity and may produce random cuts in the DNA. Restriction digestion diagram Restriction Digestion: Other Considerations Do both enzymes work at the same temperature? The majority of restriction enzymes work best at 37°C, but those isolated from thermophilic bacteria require higher temperatures for maximal activity (e.g., BstXI and BstZI work best at 50°C). Some enzymes work best below 37°C, like SmaI (25°C) and CspI (30°C). If you must work with two enzymes with different optimum temperatures, you can use the sequential digest method. Assemble all components, perform the lower-temperature digest first, and then digest at the higher temperature. Usually an hour at each temperature will work fine. When working with an enzyme that requires a temperature above 37°C, evaporation of the reaction can lead to increased glycerol concentration, which can in turn lead to star activity. Evaporation can be avoided in such reactions by applying a few drops of molecular biology grade mineral oil above the reaction. Clean up with the Wizard® SV Gel and PCR Clean-Up System to remove the mineral oil and recover the pure DNA. Do my enzymes exhibit methylation sensitivity? An often-overlooked reason for restriction enzyme digest failure is sensitivity to Dam and Dcm methylation. Many common bacterial strains like JM109, XL1-Blue and DH5α™ are positive for these two genes. The dam gene encodes a DNA adenosine methylase that methylates the N6 position of the adenine residue in the sequence: 5´…GATC…3´, a common sequence within many restriction sites. The dcm gene encodes a DNA cytosine methylase that methylates the C5 position of the internal cytosine residue in the sequence: 5´…CCAGG…3´. Some restriction enzymes are sensitive to these methylations and will not cut their recognition sequence if the methylation occurs within the recognition site (e.g., BclI and Dam methylation) or overlaps the recognition site (e.g., the ATCGAT recognition site falling within the context of …GATCGAT… or …ATCGATC…for Dam methylation). Need to digest a piece of DNA with a Dam or Dcm methylase-sensitive enzyme? Check to see if the enzyme has an isoschizomer or neoschizomer. The isoschizomer or neoschizomer may not be sensitive to the methylation. Transform the plasmid into a dam/dcm minus bacterial strain like JM110 Double Enzyme Digests Double Digests with a Common Buffer Restriction enzymes are supplied with the most compatible buffer. For double-digests a different buffer may be appropriate for maximal activity of both enzymes. In these cases, activities in other buffers must be assessed by consulting buffer activity charts. The Restriction Enzyme Resource includes information on compatibility of restriction enzymes with various Promega buffers. The Promega Restriction Enzyme tool also provides functionality to help match enzymes with the most compatible buffer for double digests. Ideally, you want to choose a buffer in which each enzyme retains at least 75%. For instance, if you were to perform a double digest with EcoRI (optimal in Buffer H) and BamHI (optimal in Buffer E) you would choose in Buffer E because the BamHI has 100% activity and EcoRI has 75–100% activity. Both enzymes will maintain acceptable levels of activity in this buffer. Double Digests without a Common Buffer Some enzymes just do not partner well. Take PstI and SpeI, for example. PstI is under optimal conditions in Buffer H, while SpeI works best in Buffer B with 100% activity. However, in Buffer B, PstI has only 50–75% activity. Three typical options are available. Sequential Method: Perform sequential digests. First digest with SpeI in Buffer B, purify DNA, and then perform the PstI digest in Buffer H. Incubate Longer: Assemble the reaction as usual in Buffer B and incubate 2–4 hours. Add More Enzyme: Add 1.5–2.0µl of PstI and incubate 1–2 hours. The sequential method seems labor-intensive, but kits like the Wizard® SV Gel and PCR Clean-Up System make the process very easy. The entire digest can be cleaned and eluted in 15µl of water. This means the buffer, enzyme and BSA can be added directly to the eluted DNA to bring the reaction to 20µl for the second optimal digest. This is really your only option if both enzymes have no compatibility (i.e., activity in buffer is less than 25%). Incubating longer or adding more enzyme may provide alternatives to performing sequential digests, depending on the enzymes involved. Longer incubation can improve the percent cleavage of the template. This is useful if both enzymes have at least 50% activity in a single buffer. Adding more enzyme can be tricky, especially if one of the enzymes is prone to star activity in higher glycerol concentrations. Remember, restriction enzymes are usually stabilized by 50% glycerol so they do not freeze in –20°C storage. Star activity may occur when the digestion glycerol concentration in the reaction rises above 5%. Partial Restriction Digests Many strategies have been employed to do partial digests including decreasing reaction temperature, using a non-optimal buffer, and decreasing units of enzyme. The method presented here uses dilutions of enzyme in the optimal buffer. The key to doing partial digests is to have a way in which you can differentiate partial digests from complete digests. In other words, your resulting products must have a discernable base pair-size difference on the agarose gel so you can cut out the correct band and perform gel isolation to purify the fragment for ligation into the destination vector. In the following example, the parent vector is first linearized, and then a partial digest is performed on the linearized vector. Digest 10µg of parent vector to completion in order to linearize using the first restriction enzyme in a 50µl reaction. Purify the linearized vector with the Wizard® SV Gel and PCR Clean-Up System directly from the digestion. Elute in 20µl nuclease-free water. On ice, create serial dilutions of to yield 5, 2.5, 1.25, 0.625, 0.313, 0.156, 0.078, 0.039U of the second restriction enzyme per 18µl of solution. Make dilutions in 1X RE Buffer containing 0.1mg/ml Acetylated BSA. Add 2µl of the purified vector to each dilution to bring the reaction volume to 20µl. Incubate all reactions at 37°C for 30–45 minutes. Add loading dye to each reaction and analyze digests by agarose gel electrophoresis. Identify and cut bands from the gel containing the DNA fragment of interest. Purify insert using the Wizard® SV Gel and PCR Clean-Up System. Elute in 15–20µl nuclease-free water. Proceed to ligation reaction. Illustration of a partial restriction digest cloning scheme More Restriction Enzyme Resources Restriction Enzyme Resource: The science behind REs, how they work, and what to be aware of as you search for the enzyme best suited to your needs. Restriction Enzyme Tool: Desktop or iOS app to look up REs by cut site, find compatible buffers or iso/neoschizomers. restriction enzyme tools Creating Blunt Ends Turning an Overhang into a Blunt End Occasionally you encounter a subcloning application where the choice of restriction sites you can use is limited or where no restriction sites exist in common between vectors and insert. Blunt-ended ligation is an option in these situations. Most vectors contain cut sites in the multiple cloning site for enzymes that make blunt cuts, like EcoRV or SmaI. However, the parent vector containing your insert may not contain a restriction site for an enzyme that makes a blunt cut. Blunting a reaction to form could be useful. Two enzymes are commonly used to generate blunt ends: T4 DNA Polymerase and the Klenow Fragment of DNA Polymerase I. Both polymerases will fill in a 5’ protruding end or remove a 3´ overhang. A third nuclease which is gaining in popularity, Mung Bean Nuclease, can remove a 5´ overhang. Blunting an Overhang using T4 DNA Polymerase T4 DNA Polymerase has excellent activity in Promega Restriction Enzyme Buffers B, C, E, and MULTI-CORE™, displaying more than 70% activity. The protocol below is for a blunting reaction following restriction digestion and has been tested with the buffers listed above. The following protocol is written to follow a 50µl digestion. The 50µl digestion is recommended to reduce the concentration of glycerol coming from both the restriction enzymes and the T4 DNA Polymerase. Reducing the glycerol concentration prevents potential star activity that may be associated with some restriction enzymes. Digest DNA (0.5–2.0µg) in a 50µl volume. Restriction digest should contain 0.1µg/µl acetylated BSA. Add 5 units of T4 DNA Polymerase/µg DNA. Add dNTPs to a final concentration of 100µM (e.g., 0.5µl of dNTP Mix [Cat.# U1511]). Incubate at 37°C for 5 minutes. Stop the reaction by adding 2µl of 0.5M EDTA. The DNA may be purified using the Wizard® SV Gel and PCR CleanUp System direct purification protocol. If purity of the product is a large concern, run the DNA on a gel to extract the desired band before Wizard® SV Gel and PCR CleanUp System purification. T4 DNA Polymerase Details Cat. M4211: 100u, 5–10u/µl Cat.# M4215: 500u, 5–10u/µl T4 DNA Polymerase has highly active exonuclease activity (200 times more active than that of DNA polymerase I). When using high concentrations of dNTPs (e.g., 100µM), degradation of the DNA will stop at the duplex DNA. However, if the dNTPs are exhausted, T4 DNA Polymerase will degrade the dsDNA. View Product Blunting an Overhang using Klenow Fragment Following the restriction enzyme digestion that generated the 5´-protruding ends, purify the DNA from the reaction with a system like the Wizard® SV Gel and PCR Clean-Up System. Assemble the following reaction: 1–4µg DNA template 2µl 10X Klenow Buffer 0.2µl Acetylated BSA (10µg/µl) 0.8µl dNTPs (1mM each) 1 unit Klenow Polymerase/µg DNA Nuclease-Free Water to 20µl Incubate at ambient room temperature for 10 minutes. Stop the reaction by incubating the mixture for 10 minutes at 75°C. The DNA may be purified using the Wizard® SV Gel and PCR CleanUp System direct purification protocol. If purity of the product is a large concern, run the DNA on an electrophoresis gel to extract the desired band before Wizard® SV Gel and PCR CleanUp System purification. Note: Promega Restriction Enzyme Buffers A, B, C, D, E and H may be substituted for the 10X Klenow Buffer, but polymerase activity is 27–43% of the 10X Klenow Buffer. DNA Polymerase I Large (Klenow) Fragment Details Cat.# M2201: 150u, 5–10u/µl Cat.# M2206: 500u, 5–10u/µl View Product Dephosphorylation of Vectors Preventing vector self-ligation is critical for reducing subcloning background. The efficiency of ligating the plasmid to itself is far better than ligating a separate piece of DNA into the vector and is the favored reaction. Removing the 5´ phosphates of the linearized vector will prevent T4 DNA Ligase from re-circularizing the vector. Calf Intestinal Alkaline Phosphatase (Cat.# M1821) is the classic enzyme for vector dephosphorylation. The enzyme can be used on 5´ recessed ends (i.e., results from an enzyme leaving a 3´ overhang), 5´ overhangs and blunt ends. After dephosphorylation, the enzyme must be removed either by direct purification, or gel electrophoresis and gel isolation with DNA purification systems like the Wizard® SV Gel and PCR Clean-Up System. TSAP Thermosensitive Alkaline Phosphatase (Cat.# M9910) can be used in place of Calf Intestinal Alkaline Phosphatase and offers the advantage of simple heat denaturation to inactivate the enzyme without the need for further purification. If the plasmid vector being used was linearized with a single restriction enzyme (generating either a blunt or overhanging end), then dephosphorylation of the vector is a prerequisite to reduce re-ligated vector background. However, if the vector was cut with two different restriction enzymes that leave incompatible ends (this does not include two different enzymes that each leave blunt ends), then dephosphorylation may be omitted. One exception to this is when the selected restriction sites lie close to one another in the vector. In this case, it is still advisable to dephosphorylate the vector, because you cannot be certain from looking at the digested plasmids on the gel if both enzymes cut the plasmid to completion. The presence of a small amount of single enzyme-cut plasmid vector in the subsequent ligation reaction can dramatically increase background, which could make it difficult to identify your desired recombinant. Gel purification of the processed destination vector before ligation ensures that uncut and partially cut vectors are removed from the subcloning reaction. Dephosphorylating Vectors: Calf Intestinal Alkaline Phosphatase (CIAP) Dephosphorylation Immediately After Restriction Digestion Add the following components directly to the digested DNA. The CIAP may be diluted on ice in 1X CIAP Buffer immediately before use. Discard any unused, diluted enzyme. 10µl CIAP 10X Reaction Buffer* 1–2µl CIAP (0.01u/pmol of ends**) Nuclease-Free Water to 100µl * The CIAP Buffer must be added to the reaction for efficient dephosphorylation. The diluted CIAP needs the Zn2+ from the buffer to work effectively. **For pmol of ends, simply multiply the pmol of DNA by 2. For example, 1µg of a 1kb DNA fragment will convert to 1.52pmol of DNA and converts to 3pmol of ends. Note: Dilution of the standard CIAP (1u/µl) is not absolutely necessary, but these are the conditions under which the enzyme is tested at Promega. Purify DNA using the Wizard® SV Gel and PCR Clean-Up System and proceed to ligation. The Biomath Calculator provides a convenient tool for calculating pmol of DNA. Dephosphorylation of Purified DNA Dilute sufficient CIAP for immediate use in CIAP 1X Reaction Buffer to a final concentration of 0.01u/µl. Each pmol of DNA ends will require 0.01u CIAP. Assemble the following reaction: 40µl DNA (up to 10pmol of ends) 5µl CIAP 10X Reaction Buffer up to 5µl diluted CIAP (0.01u/µl) Nuclease-Free Water to 50µl Note: Dilution of the standard CIAP (1u/µl) is not absolutely necessary, but these are the conditions under which the enzyme is tested at Promega. Incubate using one of the following conditions, depending on the type of ends present: 5´ Overhangs: Incubate for 30 minutes at 37°C, add another 0.01u/pmol of ends of CIAP and repeat incubation. 3´ Overhangs or Blunt Ends: Incubate for 15 minutes at 37°C then for 15 minutes at 56°C. Add another 0.01u CIAP/pmol ends and repeat incubations at both temperatures. Purify DNA using the Wizard® SV Gel and PCR CleanUp System and proceed to ligation. Alkaline Phosphatase, Calf Intestinal Cat.# M1821: 1,000u, 1u/µl Cat.# M2825: 1,000u, 20u/µl View Product Ligation Molecular biologists have exploited DNA ligases to insert pieces of DNA into vectors for decades. The enzyme most commonly used is derived from bacteriophage T4. T4 DNA Ligase is about 400-fold more active than E. coli DNA ligase for ligating blunt ends, and thus is the enzyme of choice for all molecular biology requirements. Promega offers T4 DNA Ligase in standard (Cat.# M1801) or high-concentrate (Cat.# M1794) form, with the standard Ligase Buffer (Cat.# C1263) or with the 2X Rapid Ligation Buffer (Cat.# C6711) offered in the LigaFast™ Rapid DNA Ligation System (Cat.# M8221). The LigaFast™ System allows rapid, 5-minute ligations for 5´ or 3´ overhang cohesive ends or 15-minute ligations for blunt ends. How Does DNA Ligase Work? DNA ligases are responsible for joining gaps that form in DNA during replication, DNA repair and recombination (Okazaki et al, 1968). DNA ligases catalyze the formation of a phosphodiester bond between adjacent nucleotides with the concomitant hydrolysis of ATP to AMP and inorganic phosphate. DNA ligases will only form this covalent linkage in a duplex molecule (e.g., at a nick in dsDNA or when joining cohesive- or blunt-ended dsDNAs (Higgins and Cozzarelli, 1989). The ligation mechanism occurs in three stages. First, is the formation of an enzyme-nucleotide intermediate through transfer of an adenylyl group (AMP) from ATP to the ε-amine group of a lysine residue in the enzyme. This results in the release of pyrophosphate from ATP. Second, the adenylyl group is transferred from the enzyme to the 5´-phosphate of the DNA, thereby activating it. Third, a phosphodiester bond is formed by nucleophilic attack of the 3´-hydroxyl group of the DNA with concomitant release of AMP. Ligase Buffers contain ATP to drive the reaction. Try to avoid multiple freeze-thaw cycles of the buffer. Dispense the buffer into smaller volumes to minimize the freeze-thaw cycles on each aliquot. Ligation Protocol: LigaFast™ Rapid DNA Ligation System We recommend starting with a 1:2 molar ratio of vector:insert DNA when cloning a fragment into a plasmid vector. The following example illustrates the conversion of molar ratios to mass ratios for a 3.0kb plasmid and a 0.5kb insert DNA fragment: 05kb-ligation Example: How much 0.5kb insert DNA should be added to a ligation in which 100ng of 3kb vector will be used? The desired vector:insert ratio will be 1:2. calculation-of-insert-vector-ratio The following example of a ligation reaction consisting of a 3kb vector and a 0.5kb insert DNA uses the 1:2 vector:insert ratio. Typical ligation reactions use 100–200ng of vector DNA. Assemble the following reaction in a sterile microcentrifuge tube: 100ng vector DNA 33ng insert DNA 5µl 2X Rapid Ligation Buffer 1µl T4 DNA Ligase (3u/µl) nuclease-free water to 10µl Incubate the reaction at room temperature for 5 minutes for cohesive-ended ligations, or 15 minutes for blunt-ended ligations. Ligation Protocol: T4 DNA Ligase We recommend using a 1:1, 1:3 or 3:1 molar ratio of vector:insert DNA when cloning a fragment into a plasmid vector. The following example of a ligation reaction consists of a 3.0kb vector and a 0.5kb insert DNA uses the 1:3 vector:insert ratio. Typical ligation reactions use 100–200ng of vector DNA. Assemble the following reaction in a sterile microcentrifuge tube: 100ng vector DNA 50ng insert 1µl Ligase 10X Buffer 1µl T4 DNA Ligase (3u/µl) Nuclease-Free Water to 10µl Incubate the reaction: 22–25°C, 3 hours Cohesive ends 4°C Overnight Cohesive ends 15°C, 4–18 hours Blunt ends Ligation Controls Controls help verify that everything is functioning normally in your subcloning reaction. If something does go wrong, you can use your controls to figure out where a problem might have occurred. When ligating insert and vector, you could include a control ligation of vector with no insert, typically referred to as the “vector only control”. Carry this reaction through transformation and plating. The number of colonies you see can be a good indicator of how well a ligation reaction performed and how many background colonies to expect on your plate. If you are concerned that your ligase may not be performing up to expectations, you can do a quick and easy test of your ligase by taking 1µg of a standard DNA digest marker (e.g., Lambda DNA Hind III Markers [Cat.# G1711]) and performing a 15 to 30-minute ligation reaction under normal conditions. Run the ligation reaction on a gel along with the standard marker you chose for your test. You should see DNA of much higher molecular weight in comparison to the marker. Alternatively, a plasmid that has been cut with a single restriction enzyme may also be used as a ligation control. This sample may then be used in transformation as a transformation control. LigaFast Rapid DNA Ligation System Cat.# M8221: 30 reactions Cat.# M8225: 150 reactions View Product T4 DNA Ligase Cat.# M1801: 100u, 1–3u/µl Cat.# M1804: 500u, 1–3u/µl Cat.# M1794: 500u, 10–20u/µl View Product Purification of Vector and Insert Purification of the insert and destination vector are absolutely critical for success in subcloning applications. Years ago, each step called for phenol:chloroform extractions followed by ethanol precipitation to remove enzymes such as calf intestinal alkaline phosphatase from enzymatic vector manipulations. Guanidine-based nucleic acid clean-up systems greatly simplified the removal of enzymes. Gel isolation methods further improved the efficiency of subcloning by segregating the wanted reactants from the unwanted reactants. Gel isolation of vector after digestion reduces background by eliminating uncut vector from the ligation. Wizard® SV Gel and PCR Clean-Up System The Wizard SV Gel and PCR Clean-Up System is designed to extract and purify DNA fragments directly from PCR or from agarose gels. Fragments of 100bp to 10kb can be recovered from standard or low-melt agarose gels (up to 3% agarose) in either Tris acetate (TAE) or Tris borate buffer (TBE). Up to 95% recovery is achieved, depending upon the DNA fragment size. This membrane-based system, which can bind up to 40µg of DNA, allows recovery of isolated DNA fragments or PCR products in as little as 15 minutes, depending on the number of samples processed and the protocol used. To make things even easier, up to 10 gel slices (3.5g total) may be processed on a single column with sequential loading. Wizard SV Gel and PCR Clean-Up System can remove ethidium bromide and tough enzymes like calf intestinal alkaline phosphatase (See Buros, M. and Betz, N. (2002) Removal of ethidium bromide and calf intestinal alkaline phosphatase using the Wizard SV Gel and PCR Clean-Up System). Samples can reliably be eluted in as little as 15µl of nuclease-free water. The purified DNA can be used for automated fluorescent sequencing, cloning, labeling, restriction enzyme digestion or in vitro transcription without further manipulation. Gel Electrophoresis Agarose Gel Electrophoresis of DNA Running double-stranded, linear DNA (like plasmid DNA from restriction enzyme digests) on an agarose gel is a routine activity in molecular biology laboratories. The basic method is very straightforward: Set up the gel electrophoresis apparatus as recommended by the manufacturer. Weigh the required amount of agarose and add it to the appropriate volume of TAE or TBE 1X Buffer in a flask or bottle. For example, to prepare a 1% agarose gel, add 1.0g of agarose to 100ml of buffer. Note: The volume of buffer and agarose should not exceed half the volume of the container. Heat the mixture in a microwave oven or on a hot plate for the minimum time required to allow all the agarose to dissolve. Interrupt the heating at regular intervals and swirl the container to mix the contents. Do not allow the solution to boil over. CAUTION: The container and contents will be hot! Swirling may also cause solution to boil vigorously. Use adequate precautions. Cool the solution to 50–60°C and pour the gel. Allow the gel to form completely (typically, 30 minutes at room temperature is sufficient). Remove the comb from the gel, place it in the electrophoresis chamber and add a sufficient volume of TAE or TBE 1X buffer to just cover the surface of the gel. Load samples with 1X Blue/Orange Loading Dye into the wells. Connect the gel apparatus to an electrical power supply and apply an appropriate voltage to the gel. Standard gel electrophoresis of DNA fragments greater than 2kb typically call for between 5 – 8 volts/cm. Higher voltages and shorter runs will decrease the resolution of the gel and may also cause overheating that can melt the agarose. After electrophoresis is complete, remove the gel for staining. Either soak it in a solution of 0.5µg/ml ethidium bromide for 30 minutes at room temperature, or in a solution of Diamond Nucleic Acid Dye diluted to 1:10,000 in the same type of buffer used to cast the gel for 15 to 30 minutes, protected from light. Note: Ethidium bromide or Diamond Nucleic Acid Dye may also be incorporated in the gel during preparation, and in the electrophoresis buffer, at the same concentration as would be used for staining. This eliminates the need for post-electrophoretic staining but may interfere with accurate size determination of DNA fragments. CAUTION: Always wear gloves when working with ethidium bromide. Place the gel on a UV lightbox (if using either stain), or on any other gel documentation system, or blue light transilluminator if using Diamond Nucleic Acid Dye. Photograph the gel according to the specification recommended for your camera. Diamond Nucleic Acid Dye may be visualized and photographed with any system that has a SYBR® Gold filter or setting. CAUTION: Use protective eyewear when working with a UV light source. Note: If using ethidium bromide, you may wish to destain or rinse the gel in fresh 1X running buffer prior to viewing it on the UV lightbox. Solution Compositions Nearly all of these reagents can be purchased premade including the agarose gels. Here are the directions if you wish to prepare your own reagents. Blue/Orange Loading Dye, 6X (Cat.# G1881) 10mM Tris-HCl, pH 7.5 50mM EDTA 15% Ficoll® 400 0.03% bromophenol blue 0.03% xylene cyanol FF 0.4% orange GOne or more dyes can be left out of the recipe to create a custom loading dye. TAE 50X Buffer (1L) (Available in a 10X or 40X solution from Promega [Cat.# V4271 and V4281, respectively]): Dissolve 242g Tris base and 37.2g disodium EDTA dihydrate in 900ml of deionized water. Add 57.1ml glacial acetic acid and adjust the final volume with water to 1 liter. Store at room temperature or 4°C. TBE 10X Buffer (1L) (Available in a 10X solution from Promega [Cat.# V4251]): Dissolve 108g of Tris base and 55g boric acid in 900ml deionized water. Add 40ml 0.5M EDTA (pH 8.0) and increase the final volume to 1L. Store at room temperature or 4°C. Diamond™ Nucleic Acid Dye Cat.# H1181: 500µl Diamond Dye is a sensitive fluorescent dye that binds to single-stranded DNA, double-stranded DNA and RNA, and is used to stain and visualize nucleic acids in gels. The dye does not require prewashing or destaining of gels and is more sensitive than ethidium bromide, so less sample nucleic acid and nucleic acid markers are required for visualization. View Product PCR Cloning You may wish to subclone your PCR product into a plasmid cloning vector. When PCR was in its infancy, researchers found that subcloning PCR products by simple blunt-ended ligation into blunt-ended plasmid cloning vectors was not easy. Part of the challenge is thermostable DNA polymerases, like Taq DNA polymerase, add a single nucleotide base extension to the 3´ end of blunt DNA in a template-independent fashion (Clark, 1988. Mole et al, 1989). These polymerases usually add an adenine, leaving an “A overhang.” Historically, researchers have used several approaches to overcome the cloning difficulties presented by the presence of A overhangs on PCR products. One method involves treating the product with the Klenow fragment of E. coli DNA Polymerase I to create a blunt-ended fragment for subcloning. However, this technique is not particularly efficient for PCR fragments. Another method commonly used by researchers is to add restriction enzyme recognition sites to the ends of the PCR primers (Scharf et al, 1986) to create recognition sites in the PCR fragment. The PCR product is then digested and subcloned into the desired plasmid cloning vector in a desired orientation. Care must be exercised in primer design when using this method, as not all REs cleave efficiently at the ends of DNA, and you may not be able to use every RE you desire (Kaufman and Evans, 1990). Some REs require extra bases outside the recognition site, adding further expense to the PCR primers as well as risk of priming to unrelated sequences in the genome. The most popular method for cloning PCR products is T-Vector cloning. In essence, the plasmid cloning vector is treated to contain a 3´T overhang to match the 3´A overhang of the amplicon (Mezei and Storts, 1989). The A-tailed amplicon is directly ligated to the T-tailed plasmid vector with no need for further enzymatic treatment of the amplicon other than the action of T4 DNA ligase. Promega has systems based on this technology for routine subcloning and direct mammalian expression. t-vector cloning illustration Illustration of T-vector cloning protocol. Ends Left on PCR Products by Thermostable Enzymes. Polymerase Type of End* Taq (and GoTaq®) DNA Polymerases 3´ A overhang Tfl DNA Polymerase 3´ A overhang Tth DNA Polymerase 3´ A overhang Pfu DNA Polymerase Blunt End Tli DNA Polymerase Blunt End Long PCR mixes Blunt End Other proofreading polymerases Blunt End *All bases may be found at 3´ overhang; adenine tends to be encountered most often. T-Vector Systems: pGEM®-T and pGEM®-T Easy Vectors The most basic need in PCR subcloning is a simple, general cloning vector. The pGEM®-T and pGEM®-T Easy Vector Systems are designed for just that purpose. The vectors provide convenient T7 and SP6 promoters that can serve as binding sites for sequencing primers, or for promoting in vitro transcription on either strand of the insert when the appropriate RNA polymerase is used. The vectors have the lacZα gene, allowing easy blue/white screening for recombinants using an appropriate bacterial strain (e.g., JM109, DH5α™, XL1 Blue, etc). The pGEM®-T Vectors are provided with 2X Rapid Ligation Buffer, which allows efficient ligation in just 1 hour with the supplied T4 DNA Ligase. You can either supply your own favorite E. coli cells or purchase the system with Promega JM109 Competent Cells. For maximum subcloning efficiency, purify the PCR product before subcloning. The presence of PCR primers and primer dimers can reduce subcloning efficiency. PCR Cloning Controls Each Promega PCR cloning system is provided with a control insert. The ligation and subsequent transformation of this positive control can give you a lot of information with regard to the ligation and transformation of your insert. The total number of blue colonies obtained with the Promega-supplied positive control insert and no-insert controls should be approximately equal. The negative control may have some white colonies as well. Interpreting Results from T-Cloning Experiments Experimental insert looks like control insert in efficiency and percent white colonies: Successful experiment. Greater than 80% of the white colonies should contain inserts. Experimental insert and control insert look like negative control: Ligation has failed. Avoid multiple freeze/thaws of the ligation buffer. Ligase buffer contains ATP and could be damaged by freeze/thaw cycles. You may need to dispense the ligase buffer into smaller aliquots for your experimental needs. No colonies with experimental insert, control insert or negative control: Transformation has failed. Reassess the competent cells with an intact, supercoiled plasmid and determine the transformation efficiency. Use cells >1 × 108 cfu/µg to ensure >100 colonies from the control insert ligation. Experimental insert has more blue colonies than control insert or negative control and fewer white colonies than control insert: In-frame insertion, no interruption of α-fragment. The pGEM®-T Vector Control DNA will produce recombinants that generate white colonies because the lacZ reading frame is interrupted. White colonies also form with successful insertion of other DNA fragments that disrupt the lacZ reading frame. Occasionally, a successfully inserted fragment does not disrupt the lacZ reading frame, leading to the growth of blue colonies. Although this tends to occur most frequently with PCR products of 500bp or less, inserts of up to 2kb may result in blue colonies. Moreover, some insert DNAs can also result in pale blue colonies or “bullseye” colonies with a blue center and a white perimeter. These are still successful insertions. Subcloning Using PCR Primers Containing Restriction Sites It’s possible that the ends of the desired insert DNA do not contain a suitable restriction enzyme site. This problem can be solved by using PCR to generate a site in an appropriate location. For this technique, the restriction enzyme site is designed into the 5´-end of the PCR primer. Because certain restriction enzymes inefficiently cleave recognition sequences located at the end of a DNA fragment, it is advisable to include at least four additional bases in front of the restriction recognition site. For the majority of restriction enzymes, this will result in efficient cleavage. Success when digesting PCR products can depend on the purity of the PCR product. Primers and primer dimers are present in overwhelming quantities when compared to the actual PCR product. Your PCR product will be competing with primers and primer dimers for the attention of the restriction enzyme, resulting in conditions favoring a partial restriction digest. After performing PCR, a simple clean-up of the reaction with the Wizard® SV Gel and PCR Clean-Up System can improve restriction enzyme cleavage. If you encounter a situation where the PCR product will not subclone, the digest may be adversely affected by proximity to the end of the PCR product. The PCR product can be subcloned into the pGEM®-T Easy Vector, which has restriction sites properly placed for efficiency. PCR Cloning Vectors: pGEM®-T Vector Systems Cat.# A3600: pGEM®-T Vector System I (you supply competent cells), 20 reactions Cat.# A3610: pGEM®-T Vector System II (supplied with High Efficiency JM109 Competent Cells), 20 reactions Cat.# A1360: pGEM®-T Easy Vector System I (you supply competent cells), 20 reactions Cat.# A1380: pGEM®-T Easy Vector System II (supplied with High Efficiency JM109 Competent Cells), 20 reaction Both the pGEM®-T and pGEM®-T Easy Vectors contain numerous restriction sites within the multiple cloning region. The pGEM®-T Easy Vector multiple cloning region is flanked by recognition sites for the restriction enzymes EcoRI, BstZI and NotI, providing three single-enzyme digestions for release of the insert. The pGEM®-T Vector cloning region is flanked by recognition sites for the enzyme BstZI. Alternatively, a double-digestion may be used to release the insert from either vector. View pGEM®-T SystemsView pGEM®-T Easy Systems Bacterial Transformation Properties of E. coli Subcloning Strains Common laboratory strains of E. coli, like JM109, DH5α™ and XL-1 Blue, are different from their wildtype counterparts. These strains carry some mutations designed to help propagate plasmids. Typically, laboratory strains have a mutation in the recA gene (recA1), a gene involved in recombination. The mutant gene limits recombination of the plasmid with the E. coli genome so that the plasmid inserts are more stable (the recA1 mutation is more effective than the recA13 mutation). Each of these strains also carries the endA1 mutation that inactivates a nuclease that might co-purify with plasmids during purification. This mutation allows for purification of higher quality plasmids. Special treatments must be performed on plasmids from strains that do not have this mutation (e.g., RR1, HB101, etc.) to eliminate the nuclease from the plasmid prep (e.g., an Alkaline Protease digestion). Common laboratory strains of E. coli are typically defined as K strains or B strains based on the presence of the restriction and modification system that functions around EcoKI or EcoBI, respectively. In a wildtype K strain, the E. coli will have both the EcoKI restriction enzyme to cleave foreign DNA and EcoKI methylase to protect and mask host DNA recognition sequences. In B strains, the EcoBI restriction enzyme and methylase serve the same purpose. Strains like JM109, DH5α™ and XL-1 Blue are K strains but carry the hsdR17 (rK–, mK+) mutation. This mutation knocks out the EcoKI restriction enzyme but leaves the methylase intact. Therefore, these strains will not degrade plasmid DNA isolated from a B or K strain but will methylate it. This is useful if the DNA must be transferred to a K strain with an intact K restriction and methylation system. If you wish to incorporate blue/white selection into your subcloning scheme, you need to transform E. coli carrying a lacZ∆. This mutation deletes a portion of the β-galactosidase gene, leaving what is termed the ω-fragment. The plasmid vector supplies this deleted portion, or α-fragment. Once inside the bacterium, the plasmid produces the α-fragment and the E. coli produces the ω-fragment, which combine to make a functional β-galactosidase. If grown on plate containing 5-bromo-4-chloro-3-indoyl-β-D-galactopyranoside (X-gal), the colony will turn blue as a result of β-galactosidase activity and indicate full complementation of the bacterium by the plasmid. This is termed α-complementation. Blue/White cloning methods use plasmids with a multiple cloning region within the coding sequence of the α-fragment. Disruption of the reading frame due to the presence of the insert will produce a non-functional α-fragment incapable of α-complementation. These disrupted plasmids are differentiated from the plasmids without insert by the color of the colony (white versus blue), hence the term blue/white selection. Strains like JM109, DH5α™ and XL-1 Blue have the necessary deletion. One difference between these strains lies in how you get the bacterium to produce the ω-fragment. Both JM109 and XL-1 Blue have a second mutation call lacIq. This mutation leads to increased production of the LacI repressor that stops transcription from the lac operon until substrate is present. To relieve this repression, these strains are grown on media containing the non-cleavable lactose analog, isopropyl-β-D-thiogalactopyranoside (IPTG). DH5α™ does not have the lacIq mutation and constantly produces a low level of the ω-fragment through leaky transcription of the lac operon and therefore does not require IPTG for blue/white selection. Ready-to-Use JM109 Competent Cells Competent cells at Promega are available in two efficiencies; High Efficiency at greater than 108cfu/µg and Subcloning Efficiency at greater than 107cfu/µg. JM109 cells are an ideal host for many molecular biology applications. For example, standard subcloning that requires blue/white screening, scale-up for large plasmid preparations, and routine minipreps. The cells are recA1, endA1, and lacZ∆M15. Cat.# L2001: JM109 Competent Cells, Cloning and Single Use, >108 cfu/µg, 5 x 200µl Cat.# L1001: JM109 Competent Cells, Subcloning, >107 cfu/µg, 5 x 200µl View ProductView Protocol Making your own competent cells Preparation of Competent Cells: Modified RbCl Method This rubidium chloride protocol gives better transformation efficiencies than the CaCl2 procedure, for most strains. The procedure is an adaptation of one described in Hanahan, D. (1985) In: DNA Cloning, Volume 1, D. Glover, ed., IRL Press, Ltd., London, 109. Materials to Be Supplied by the User LB medium and plates LB + 20mM MgSO4 TFB1, ice-cold TFB2, ice-cold dry ice/isopropanol bath or liquid nitrogen Inoculate 2.5ml of LB medium in a plating tube with a single colony from an LB plate. For JM109, use M9 + B1 plate so that the F´ episome is maintained. Incubate the tube overnight at 37°C with shaking (approximately 225rpm). Subculture the overnight culture at a 1:100 dilution. Inoculate 250ml of LB supplemented with 20mM MgSO4 in a 1L flask, with 2.5ml of the overnight culture. Grow the cells at 37°C with shaking until the OD600 reaches 0.4–0.6 (usually 5–6 hours, but the time may vary). Pellet the cells by centrifugation at 4,500 × g for 5 minutes at 4°C. For a 250ml culture, use two 250ml centrifuge bottles in a large rotor; half of the culture in one bottle, half in the other. Be sure to balance the bottles. Gently resuspend the cell pellet in 0.4 of the original volume of ice-cold TFB1. For a 250ml subculture, use 100ml total of TFB1 (50ml/bottle centrifuged). Combine the resuspended cells in one bottle. For the remaining steps, keep the cells on ice and chill all pipettes, tubes and flasks. Incubate the resuspended cells on ice for 5 minutes at 4°C. Pellet the cells by centrifugation at 4,500 × g for 5 minutes at 4°C. Gently resuspend the cells in 1/25 original volume of ice-cold TFB2. For a 250ml subculture, use 10ml of TFB2. Incubate the cells on ice for 15–60 minutes, and then dispense 100µl/tube for storage at –70°C. During dispensing, keep the cells as cold as possible. Flash-freeze the tubes in a dry ice/isopropanol bath or liquid nitrogen, and store at -70°C. JM109 competent cells prepared by this method are stable for 1 year if never thawed. Note: Competent cells may be conveniently quick-frozen using ice bath racks, or ChillBlock™. Set up an ice bath and an ethanol bath. Label the tubes on their lids. Open the labeled tubes and place them in a rack in the ice bath. Dispense 100µl cells per tube, then close the tubes. Add dry ice to the ethanol bath, wait for it to stop bubbling, and then transfer the rack and tubes to the dry ice bath for about 15 seconds. Regular ice may be used in the ethanol bath if dry ice is not available, but flash freezing will not occur. Transfer the tubes to their storage box and place in a –70°C freezer. Do not get alcohol on the lips of the tubes. Liquid nitrogen also can be used for flash-freezing, but be sure it is compatible with the rack you are using. Use only plasticware designed for liquid nitrogen. Note: Be careful not to get alcohol on the labels because it will remove them. Many E. coli strains carry episomes (e.g., F´ and P2) expanding the capabilities of the bacterium for use in subcloning applications. For example, the XL1-Blue and JM109 strains carry the lacIq∆M15 mutation on the F´ episome. The episomes are extrachromosomal replicating plasmids with a selectable marker. When making competent cells from strains with episomes, the bacteria must first be plated on selective media. For XL1-Blue, colonies are selected on tetracycline plates because the episome contains the tetR gene. This means that the strain cannot be used with subcloning plasmids containing the tetR gene for selection. JM109 cells should be selected first on M9 minimal media containing thiamine (vitamin B1). The bacterial chromosome lacks the biosynthetic genes for proline synthesis (proAB) but the episome carries those genes. Colonies grown on the M9 + B1 plates (recipe on page 48) can then be processed into competent cells ready for blue/white selection. Determining Transformation Efficiency of Competent Cells This is a general protocol for use with the procedure for producing competent cells provided above. Please follow manufacturers’ instructions when using purchased competent cells. Keep in mind, transforming more than 10ng of DNA from a ligation reaction may actually decrease transformation efficiency. Thaw a 100µl aliquot of competent cells on ice. Transfer 100µl of the cells to a 17 × 100mm polypropylene tube prechilled on ice. Add 0.1ng of a supercoiled plasmid [e.g., pGEM®-3Zf(+) Vector] in a 10µl volume to the competent cells and gently mix by swirling the pipet tip (do not mix by pipetting). Transfer the tubes from ice to a 42°C water bath and heat shock for 45–60 seconds. Place on ice immediately to cool for at least 2 minutes. Add 890µl of SOC medium (giving a concentration of 0.1ng DNA/ml) and incubate for 45 minutes at 37°C with shaking (~150rpm). Dilute cells by transferring 100µl of cells to 900µl of SOC medium (giving a concentration of 0.01ng DNA/ml). Plate 100µl of diluted cells onto LB plates with the appropriate antibiotic. You may wish to plate 100µl of undiluted cells for determining efficiency as well. Incubate the plates overnight in a 37°C incubator and count the number of colonies obtained. Transforming Ligation Reactions This is a general protocol for use with the procedure for producing competent cells provided above. Please follow manufacturers’ instructions when using purchased competent cells. Thaw a 100µl aliquot of competent cells on ice. Transfer 100µl aliquot of the competent cells to a 17 × 100mm polypropylene tube prechilled on ice. Add no more than 10ng of DNA in a maximum of 10µl from a ligation reaction to the cells and gently swirl the pipet tip to mix (do not mix by pipetting). Incubate on ice for 30 minutes. Transfer the tubes from ice to a 42°C water bath and heat-shock for 45–60 seconds. Place on ice immediately to cool for 2 minutes. Add 1ml of LB or SOC medium and incubate for 45 minutes at 37°C with shaking (~150rpm). Plate 100–200µl of the transformation mix or an appropriate dilution, onto selection plates. If you suspect low ligation efficiency, take the remaining cells and pellet by a quick 10–20 second spin in a microcentrifuge. Pour off media and resuspend pellet in about 200µl of SOC and plate. 200cfu = 2 × 105cfu/ng = 2 × 108cfu/µg DNA Transformation Controls Controls help you figure out where things may have gone wrong with the subcloning procedure. When transforming bacteria with your subcloning reaction DNA, also determine transformation efficiency. Transforming a ligation control of cut, dephosphorylated vector without insert can tell you how many background colonies you can expect in your actual vector + insert ligation. Screening for Recombinants Now that you’ve transformed your DNA and allowed the colonies to grow overnight, you need to determine if they contain the insert of interest. You can either screen them by colony PCR, or the more traditional plasmid miniprep followed by restriction digestion. Colony PCR is the most rapid initial screen. A plasmid miniprep will take an extra day to grow up the culture but will provide a lot of material for further analysis. Some researchers choose to do both but only perform minipreps on colonies identified through colony PCR to contain the insert of interest. Colony PCR Colony PCR involves lysing the bacteria and amplifying a portion of the plasmid. If your subcloning scheme will not maintain the orientation of the insert, you can even use colony PCR to screen for orientation. You can use either insert-specific primers or vector-specific primers to screen for recombinant plasmids. Simply combine a vector-specific primer with an insert-specific primer. PCR cloning using the A-overhangs left by Taq DNA Polymerase and an appropriately T-tailed vector (e.g., pGEM®-T Easy Vector) is not a technique that will retain orientation. The orientation can be rapidly assessed with colony PCR using vector-specific primers and insert-specific primers. For example, this technique was used for screening the orientation of a 1.8kb insert into the pGEM-T Easy Vector. Colony PCR was performed with the T7 Promoter Primer and either the insert-specific forward or reverse PCR primer. Eight white colonies were chosen from the cloning experiment for analysis. Clones with the T7 orientation will only produce a fragment with the T7 primer and reverse PCR primer, and clones in the opposite (SP6) orientation will only produce a fragment with the forward PCR primer as illustrated below. colony pcr illustration Colony PCR to determine cloned insert orientation Determining insert presence and orientation by colony PCR. Colonies were suspended in 50µl sterile water, boiled for 10 minutes, centrifuged at 16,000 × g for 5 minutes, and 5µl of the supernatant was used in each amplification. The DNA was amplified by PCR in 50µl volumes with 50pmol of each primer and 1.25 units of Taq DNA Polymerase. After an initial denaturation of 2 minutes at 94°C, the amplification profile was 35 cycles of denaturation (94°C for 30 seconds), annealing (55°C for 1 minute) and extension (72°C for 2.5 minutes); PCR was concluded with 1 cycle of 72°C for 10 minutes. Amplification products (8µl) were analyzed on a 1% agarose gel containing ethidium bromide. Colony Prep for Colony PCR Pick a well-isolated colony and transfer to 50µl of sterile water. Part of the colony may be transferred to LB medium containing the appropriate antibiotic for overnight culture and miniprep, if desired. Boil the colony in sterile water for 10 minutes. Centrifuge at 16,000 x g for 5 minutes. Use 5µl of the supernatant in a 50µl PCR. Screening by Plasmid Miniprep and Restriction Enzyme Digest The classic method for screening colonies involves performing a plasmid miniprep followed by restriction digestion. Well-isolated colonies are picked from a plate and transferred to culture medium containing the appropriate antibiotic for selection. Proper sterile technique is important. Many different culture media formulations are commonly used for minipreps. Promega recommends LB medium supplemented with antibiotics for miniprep cultures. If a very rich medium like Terrific Broth is used, the bacteria can grow to very high cell densities and deplete the antibiotic. Once the antibiotic is depleted, the selection pressure to keep the plasmid is removed, and the plasmid may be lost. You can inoculate the colony into 1–10ml of culture medium. If using a high-copy plasmid, 1–5ml (more typically, 1–2ml) is plenty. If you are using a low-copy plasmid, inoculate 10ml. Aerating the culture is very important for maximum cell density. A 17 × 100mm culture tube is fine for 1–2ml. If growing a larger volume, a 50ml sterile, disposable culture tube is better. Incubate the culture overnight (12–16 hours) with shaking (~250rpm). Remember, the greater the surface area, the greater the aeration. You can even grow miniprep cultures in sterile 25–50ml Erlenmeyer flasks. Once the DNA is purified, a portion of the plasmid is screened by restriction digestion. For high-copy plasmids, you can obtain 4–10µg plasmid DNA per purification (1–5ml). For low-copy plasmids, you will obtain 1–3µg plasmid DNA per purification (10ml). Use 0.5–1µg of plasmid in your digest. Design the digest so that you can easily determine if your plasmid contains insert. Note: Be sure to run uncut plasmid on the same gel for comparison. Media and Solutions LB medium (1 liter) 10g tryptone 5g yeast extract 5g NaCl Adjust pH to 7.5 with NaOH and autoclave. LB plates (1 liter) Add 15g agar to 1 liter of LB medium. Adjust to pH 7.5 with NaOH. Autoclave. Pour 15–20ml of medium into 85mm petri dishes. If necessary, flame the surface of the medium with a Bunsen burner to remove bubbles. LB medium plus antibiotic Allow the medium to cool to 55°C before adding antibiotic (either ampicillin, 125µg/ml final concentration; tetracycline,12.5µg/ml final concentration; or chloramphenicol, 20µg/ml final concentration). LB plates plus antibiotic (1 liter) Add 15g agar and a stir bar to 1 liter of LB medium. Adjust to pH 7.5 with NaOH. Autoclave. Allow to cool to ~55°C (you will be able to hold the flask for at least 10 seconds), and then add antibiotic to the appropriate final concentration. Mix the medium by stirring on a stir plate for 2–3 minutes. Pour 15–20ml of medium into 85mm petri dishes. If necessary, flame the surface of the medium with a Bunsen burner to remove bubbles. SOC medium 2.0g tryptone 0.5g yeast extract 1ml 1M NaCl 0.25ml 1M KCl 1ml Mg2+ stock (1M MgCl2 • 6H2O, 1M MgSO4 • 7H2O) filter-sterilized 1ml 2M glucose, filter-sterilized Add tryptone, yeast extract, NaCl and KCl to 97ml distilled water. Stir to dissolve. Autoclave and cool to room temperature. Add 2M Mg2+ stock and 2M glucose stock, each to a final concentration of 20mM. Adjust to pH 7.0. Filter the complete medium through a 0.2µm filter unit. M9 + B1 plates 6g Na2HPO4 3g KH2PO4 0.5g NaCl 1g NH4Cl 15g Agar Add deionized water to approximately 1L. Adjust to pH 7.4 with 10N NaOH. Autoclave and cool to ~55°C. Then add the following sterile solutions: 2.0ml 1M MgSO4 0.1ml 1M CaCl2 10.0ml 20% glucose (filter-sterilized) 1.0ml 1M thiamine-HCl (filter-sterilized) Pour 15–20ml of medium into 85mm petri dishes. TFB1 30mM potassium acetate 10mM CaCl2 H2O 50mM MnCl2 H2O 100mM Rubidium Chloride (RbCl) 15% glycerol Adjust pH to 5.8 with 1M acetic acid. Be very careful as you approach 5.8; if the pH drops below 5.8, a black precipitate may form. Filter sterilize (0.2µM) and store at room temperature. TFB2 100mM MOPS or PIPES (pH 6.5) 75mM CaCl2 10mM Rubidium Chloride (RbCl) 15% glycerol Adjust the pH to 6.5 with 1M KOH. Filter sterilize (0.2µM) and store at room temperature. Ampicillin Stock Solution Dissolve at 50mg/ml in water, filter sterilize, store in aliquots at –20°C. IPTG stock solution (0.1M) 1.2g IPTG (Cat.# V3951) Add water to 50ml final volume. Filter-sterilize and store at 4°C. X-Gal stock solution (2ml) 100mg X-gal (Cat.# V3941) Dissolve at 50mg/ml in N,N′-dimethyl-formamide. Cover with aluminum foil and store at –20°C. LB plates with ampicillin/IPTG/X-Gal Add 15g agar to 1 liter of LB medium. Autoclave. Allow the medium to cool to ~55°C before adding ampicillin to a final concentration of 100µg/ml, and then supplement with IPTG to 0.5mM and X-Gal to 80µg/ml. Pour 15–20ml of medium into 85mm petri dishes. Let the agar solidify. Store at 4°C for up to 1 month or at room temperature for up to 1 week. Related Products Bacterial Strains and Competent Cells Molecular Biology Enzymes and Reagents Molecular Weight Markers PCR Cloning Restriction Enzymes Taq Polymerase References Clark, J.M. (1988) Novel non-template nucleotide addition reactions catalyzed by procaryotic and eucaryotic DNA polymerases. Nucl. Acids Res. 16, 9677–86. Higgins, N.P. and Cozzarelli, R. (1989) In: Recombinan DNA Methodology Wu, R., Grossman, L. and Moldave, K., (Eds). Academic Press, Inc., San Diego, California. Kaufman, D.L. and Evans, G.A. (1990) Restriction endonuclease cleavage at the termini of PCR products. BioTechniques 9, 304–6. Mezei, L.M. and Storts, D.R. (1994) Cloning PCR Products. In: PCR Technology Current Innovations. Griffin, H.G. and Griffin, A.M. (eds). CRC Press, 21–7. Mole, S.E., Iggo, R.D. and Lane, D.P. (1989) Using the polymerase chain reaction to modify expression plasmids for epitope mapping. Nucl. Acids Res. 17, 3319. Okazaki, R. et al. (1968) Proc. Natl. Acad. Sci. USA 59, 598. Scharf, S.J., Horn, G.T. and Erlich, H.A. (1986) Direct cloning and sequence analysis of enzymatically amplified genomic sequences. Science 233, 1076–8.